B.C. Clark
Ohio Musculoskeletal and Neurological Institute (OMNI), Department of Biomedical Sciences, and the Division of Geriatric Medicine at Ohio University, Athens, Ohio, USA
Corresponding author: Brian C. Clark, Ph.D. Ohio University, Ohio Musculoskeletal & Neurological Institute (OMNI), 250 Irvine Hall, Athens, OH 45701, USA, Phone: 740.593.2354, Email: clarkb2@ohio.edu
J Frailty Aging 2018;in press
Published online October 22, 2018, http://dx.doi.org/10.14283/jfa.2018.35
Abstract
Abstract: Sarcopenia was originally conceptualized as the age-related loss of skeletal muscle mass. Over the ensuing decades, the conceptual definition of sarcopenia has changed to represent a condition in older adults that is characterized by declining muscle mass and function, with “function” most commonly conceived as muscle weakness and/or impaired physical performance (e.g., slow gait speed). Findings over the past 15-years, however, have demonstrated that changes in grip and leg extensor strength are not primarily due to muscle atrophy per se, and that to a large extent, are reflective of declines in the integrity of the nervous system. This article briefly summarizes findings relating to the complex neuromuscular mechanisms that contribute to reductions in muscle function associated with advancing age, and the implications of these findings on the development of effective therapies.
Key words: Sarcopenia, dynapenia, aging, muscle, strength, weakness.
Sarcopenia was originally conceptualized, thirty years ago, as the age-related loss of skeletal muscle mass (1). Over the ensuing decades, sarcopenia has come to be conceptually defined as a condition in older adults that is characterized by declining muscle mass and function, with “function” most commonly conceived as muscle weakness and/or impaired physical performance (e.g., slow gait speed) (2, 3).
The central tenet for the evolution of the definition of sarcopenia is based on the premise that the loss of muscle mass leads to the loss of muscle function (e.g., weakness) and that this contributes to limitations in physical function and mobility. Two critical arguments, however, strongly question the scientific premise of this tenet:
1. Longitudinal data indicate that the age-related changes in strength are not due to muscle wasting (4, 5), and that strength, but not mass, is associated with negative health outcomes (6, 7). For instance, using data from the Health ABC study, Delmonico and colleagues (2009) assessed changes in thigh muscle size using computed tomography and isokinetic leg extensor strength serially over a 5-year period in a cohort of older adults that were between 70-79 years at baseline (4). They reported that annualized decreases in muscle strength were 2-5 times greater than the loss of muscle size in those who lost or maintained weight over the five-year period. Moreover, individuals that gained weight actually exhibited a small increase in muscle size, but this increase in muscle size did not prevent a loss of strength. Findings of this nature clearly indicate that the loss of muscle strength (and presumably power) in older adults is modestly associated with the loss of muscle mass or size, and suggest that neurological and non-muscle mass related factors are critical in the development of age-related muscle weakness. It should be noted that muscle atrophy should not be regarded as a negligible corollary of aging. Low muscle mass is associated with negative outcomes in a variety of disease conditions, and its importance to overall health should not be diminished (8).
2. Impairments in neural activation of skeletal muscle is (9) a key contributor to muscle weakness in older adults. Grip strength, due to widespread availability of grip dynamometers and ease of assessment, is by far the most common index of muscle strength in the field of aging systems and geriatrics. Loss of grip strength with advancing age has been shown to have predictive power in relation to a range of health-related conditions (6, 7, 10, 11). Grip strength is generally interpreted as a simple measure of skeletal muscle function, which is why it has largely been used in the recent conceptual definitions of sarcopenia. The interpretation of grip strength as a measure of skeletal muscle function is, however, arguably incorrect (9). Rather, a strong case can be made that grip strength, and age-related changes in grip strength in particular, is neither simple nor a measure skeletal muscle function per se (see reference (9) for a more detailed discussion) . Instead, evidence suggests that the force generated during a maximum voluntary grip force task is around half of what would be expected if the skeletal musculature itself were fully activated by the nervous system (Figure 1) (12-14), due to reduced neural drive to the muscles (15). Specifically, the maximum force that can be produced by each finger decreases in proportion to the number of other fingers that are engaged simultaneously, such that when four fingers contribute to the grip task, the maximum force that can be generated by each digit is typically less than half that produced when it is engaged in isolation (i.e., there is a force deficit) (14, 16). Moreover, this grip strength ‘force deficit’ is larger in older adults in comparison to young adults (14, 17). In agreement with the above-mentioned notion of impairments in neural activation being a key contributor to age-related changes in muscle strength, we have reported that weaker older adults exhibit a 20% deficit in voluntary (neural) activation of the wrist flexor muscles (18). In this study, the motor nerve was electrically stimulated during a maximal voluntary wrist flexion contraction and any increment in force evoked by a stimulus indicates that voluntary activation is less than 100%. Thus, voluntary activation represents the proportion of maximal possible force that is produced voluntarily, and impairment indicates some motor units are not recruited or are not firing fast enough to produce fused contractions (19). Accordingly, these findings indicate that impairments in neural activation, broadly speaking, is a key contributor to muscle weakness in older adults.
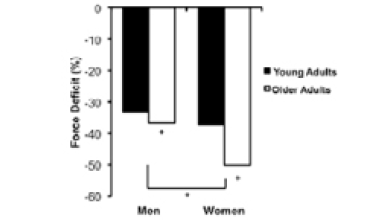
Figure 1
The force that can be generated during a maximum voluntary grip force task is around half of what would be expected if the skeletal musculature itself were fully activated. This data, which was recreated from data presented in Shinohara et al. (14), was obtained from 12 young (filled bars) and 12 older adults (unfilled bars). Subjects performed single-finger maximal voluntary contractions (MVC) as well as a four finger MVC by pressing on individual force transducers. Note the dramatic drop in the force of individual fingers during four-finger MVC tasks compared with single-finger MVC tasks (i.e., a force deficit). Further note that this force deficit was larger in older adults than young adults. Findings of this nature suggest that grip strength is heavily reflective of nervous system function, and not skeletal muscle function per se
Significant differences for men vs. women, *P<0.05 and for elderly vs. young, +P<0.05.
Collectively, findings of this nature question the notion that 1) the loss of muscle mass is a critical mechanism leading to loss of muscle strength, and 2) that age-related muscle weakness is solely due to declining skeletal muscle function per se. Rather, these findings suggest that the nervous system, and specifically the neural control of skeletal muscle, is a key contributor to declining muscle and physical function commonly observed with advancing age. There is strong proof-of-concept evidence that aging results in a plethora of changes in the neuromuscular system that could theoretically effect neuromuscular function. These include changes in the nervous system, such as reductions in corticospinal excitability, degeneration as well as altered biophysical and behavioral characteristics of motor neurons, among others (for review see (19-22)). It should also be noted there are a number of non-mass dependent age-related changes in skeletal muscle properties that may also contribute to impaired neuromuscular function (e.g., excitation-contraction uncoupling and alterations in musculotendinous properties that lead to reductions in intrinsic muscle quality) (for review see (23, 24)). There has been much discussion in the literature about the relationship between fat infiltration in muscle and dynapenia. While it’s contribution to weakness is not fully understood, there is strong evidence that questions the contributing role of intermuscular fat. Specifically, a study that tracked 1,678 older adults over a 5-year period and examined the relationship between changes in muscle size, muscle fat infiltration and muscle strength, it was observed that the change in intermuscular fat explained less than 1% of the between subject variance in the change in muscle strength (4). Nevertheless, the salient point is that strong consideration needs to be given to the multiple mechanisms contributing to age-related reductions in neuromuscular function in the development of an operational definition of sarcopenia (or dynapenia, which we have previously recommended for consideration as an alternative to the term “sarcopenia”, in order to distinguish between the age-related loss of muscle strength (dynapenia) and the age-related loss of muscle mass (sarcopenia) for the reasons stated above (20, 21)).
Are We Barking Up the Wrong Tree? Sarcopenia is commonly conceptualized as a condition of the muscular system based on the rationale that the muscular system is responsible for the function of mobility (2, 3). However, this conceptualization does not give sufficient consideration to “muscle function” being a subset of “motor function”. Accordingly, one must raise the question of whether the sarcopenia field is at a critical junction in need of a major paradigm shift away from the traditional “skeletal muscle centric” focus that the field has largely pursued. For instance, the “graying of the nation” has resulted in a large number of pharmaceutical companies pursuing compounds to enhance muscle and physical function in older adults (25). To date, they have focused on compounds designed to target skeletal muscle, such as those designed to promote muscle growth, or— at a minimum— attenuate atrophy (e.g., myostatin-inhibitors) or those designed to increase skeletal muscle calcium sensitivity. These trials have, generally speaking, reported modest, if not disappointing effects, for enhancing muscle strength and physical function. Is it possible that these disappointing results are due to these compounds targeting the entirely wrong system— skeletal muscle— as opposed to the nervous system? There has certainly been an increased interest in the role of the nervous system in muscle weakness and mobility limitations in older adults in recent years, and in the coming years this answer to this question should become clearer.
Grant Support: This work was supported, in part, by a grant from the National Institute on Aging (R01AG044424).
Conflict of Interest Statement: Brian Clark has received research funding from the National Institutes of Health, Regeneron Pharmaceuticals, Astellas Pharma Global Development, Inc., RTI Health Solutions, Ohio Department of Higher Education, and the Osteopathic Heritage Foundations. In the past 5-years Brian Clark has received consulting fees from Regeneron Pharmaceuticals, Abbott Laboratories, and the Gerson Lehrman Group. Additionally, Brian Clark is co-founder with equity and scientific director of AEIOU Scientific, LLC.
Acknowledgements: The author wishes to thank Leatha A. Clark, DPT, MS and David W. Russ, PT, PhD for providing critical comments on an initial draft of the manuscript.
Open Access: This article is distributed under the terms of the Creative Commons Attribution 4.0 International License (http://creativecommons.org/licenses/by/4.0/), which permits use, duplication, adaptation, distribution and reproduction in any medium or format, as long as you give appropriate credit to the original author(s) and the source, provide a link to the Creative Commons license and indicate if changes were made.
References
1. Clegg A, Young J, Iliffe S, Rikkert MO, Rockwood K. Frailty in elderly people. 1. Rosenberg, I.H. Summary comments. Am J Clin Nutr 1989; 50: p. 1231-1233.
2. Fielding, R.A., B. Vellas, W.J. Evans, S. Bhasin, J.E. Morley, A.B. Newman, et al. Sarcopenia: an undiagnosed condition in older adults. Current consensus definition: prevalence, etiology, and consequences. International working group on sarcopenia. J Am Med Dir Assoc 2011; 12: p. 249-56.
3. Cruz-Jentoft, A.J., J.P. Baeyens, J.M. Bauer, Y. Boirie, T. Cederholm, F. Landi, et al. Sarcopenia: European consensus on definition and diagnosis: Report of the European Working Group on Sarcopenia in Older People. Age Ageing 2010; 39: p. 412-23.
4. Delmonico, M.J., T.B. Harris, M. Visser, S.W. Park, M.B. Conroy, P. Velasquez-Mieyer, et al. Longitudinal study of muscle strength, quality, and adipose tissue infiltration. Am J Clin Nutr 2009; 90: p. 1579-85.
5. Legrand, D., B. Vaes, C. Mathei, W. Adriaensen, G. Van Pottelbergh and J.M. Degryse. Muscle strength and physical performance as predictors of mortality, hospitalization, and disability in the oldest old. J Am Geriatr Soc 2014; 62: p. 1030-8.
6. Newman, A.B., V. Kupelian, M. Visser, E.M. Simonsick, B.H. Goodpaster, S.B. Kritchevsky, et al. Strength, but not muscle mass, is associated with mortality in the health, aging and body composition study cohort. J Gerontol A Biol Sci Med Sci 2006; 61: p. 72-7.
7. Metter, E.J., L.A. Talbot, M. Schrager and R. Conwit. Skeletal muscle strength as a predictor of all-cause mortality in healthy men. J Gerontol A Biol Sci Med Sci 2002; 57: p. B359-65.
8. Wolfe, R.R. The underappreciated role of muscle in health and disease. Am J Clin Nutr 2006; 84: p. 475-82.
9. Carson, R.G. Get a grip: Individual variations in grip strength are a marker of brain health. Neurobiol Aging In Press.
10. Seidel, D., C. Brayne and C. Jagger. Limitations in physical functioning among older people as a predictor of subsequent disability in instrumental activities of daily living. Age Ageing 2011; 40: p. 463-9.
11. Bohannon, R.W. Hand-grip dynamometry predicts future outcomes in aging adults. J Geriatr Phys Ther 2008; 31: p. 3-10.
12. Li, Z.M., M.L. Latash, K.M. Newell and V.M. Zatsiorsky. Motor redundancy during maximal voluntary contraction in four-finger tasks. Exp Brain Res 1998; 122: p. 71-8.
13. Li, Z.M., M.L. Latash and V.M. Zatsiorsky. Force sharing among fingers as a model of the redundancy problem. Exp Brain Res 1998; 119: p. 276-86.
14. Shinohara, M., S. Li, N. Kang, V.M. Zatsiorsky and M.L. Latash. Effects of age and gender on finger coordination in MVC and submaximal force-matching tasks. J Appl Physiol (1985) 2003; 94: p. 259-70.
15. Ohtsuki, T. Inhibition of individual fingers during grip strength exertion. Ergonomics 1981; 24: p. 21-36.
16. Kinoshita, H., S. Kawai and K. Ikuta. Contributions and co-ordination of individual fingers in multiple finger prehension. Ergonomics 1995; 38: p. 1212-30.
17. Shinohara, M., M.L. Latash and V.M. Zatsiorsky. Age effects on force produced by intrinsic and extrinsic hand muscles and finger interaction during MVC tasks. J Appl Physiol (1985) 2003; 95: p. 1361-9.
18. Clark, B.C., J.L. Taylor, S.L. Hong, T.D. Law and D.W. Russ. Weaker Seniors Exhibit Motor Cortex Hypoexcitability and Impairments in Voluntary Activation. J Gerontol A Biol Sci Med Sci 2015; 70: p. 1112-9.
19. Clark, B.C. and J.L. Taylor. Age-Related Changes in Motor Cortical Properties and Voluntary Activation of Skeletal Muscle. Current aging science 2011.
20. Clark, B.C. and T.M. Manini. Sarcopenia =/= dynapenia. J Gerontol A Biol Sci Med Sci 2008; 63: p. 829-34.
21. Manini, T.M. and B.C. Clark. Dynapenia and Aging: An Update. The journals of gerontology. Series A, Biological sciences and medical sciences 2011.
22. Enoka, R.M., E.A. Christou, S.K. Hunter, K.W. Kornatz, J.G. Semmler, A.M. Taylor, et al. Mechanisms that contribute to differences in motor performance between young and old adults. J Electromyogr Kinesiol 2003; 13: p. 1-12.
23. Russ, D.W., K. Gregg-Cornell, M.J. Conaway and B.C. Clark. Evolving concepts on the age-related changes in “muscle quality”. Journal of cachexia, sarcopenia and muscle 2012.
24. Narici, M.V. and N. Maffulli. Sarcopenia: characteristics, mechanisms and functional significance. Br Med Bull 2010; 95: p. 139-59.
25. Garber, K. No longer going to waste. Nat Biotechnol 2016; 34: p. 458-61.