A. Medoro1, G. Scapagnini1, S. Davinelli1
1. Department of Medicine and Health Sciences «V. Tiberio», University of Molise, Campobasso, Italy
Corresponding Author: Sergio Davinelli, Department of Medicine and Health Sciences “V. Tiberio” University of Molise Via F. De Sanctis, s.n.c., Campobasso 86100, Italy, E-mail: sergio.davinelli@unimol.it, Telephone number: + 39 0874 404771
J Frailty Aging 2024;13(4)432-440
Published online October 6, 2024, http://dx.doi.org/10.14283/jfa.2024.73
Abstract
BACKGROUND: Experimental evidence suggests that polyphenols, a large group of phytochemicals found in fruits and vegetables, may preserve muscle mass and strength by increasing the expression of anabolic factors and enhancing mitochondrial function.
OBJECTIVES: This systematic review and meta-analysis aims to summarize the evidence about the effect of polyphenol supplementation on muscle mass, muscle strength, and physical performance in individuals with sarcopenia.
Methods: A systematic search was conducted using three databases (PubMed/Medline, Scopus, and Web of Science) from the date of inception to April 2024. Interventional studies examining the effect of polyphenol supplementation on muscle measures and physical performance in middle-aged and older subjects with sarcopenia were included.
Results: Of the 344 articles screened, 7 articles were included in the systematic review. Five of the 7 included studies were meta-analyzed, involving a total of 227 patients with sarcopenia. The results showed a statistically significant effect of polyphenols on muscle mass (SMD = 1.50; 95% CI: 0.26, 2.75; Z = 2.36; P = 0.02), no effect on muscle strength (SMD = 0.03; 95% CI: −0.24, 0.30; Z = 0.20; P = 0.84), and a near-significant trend on physical performance (SMD = 0.52; 95% CI: −0.03, 1.07; P = 0.06).
Conclusions: Based on the available data, this study provides pooled evidence that treatment with polyphenols may have a beneficial effect on muscle mass in sarcopenic subjects.
However, further studies with larger sample sizes are required to substantiate this effect and draw more accurate conclusions.
Key words: Polyphenols, sarcopenia, muscle mass, muscle strength, physical performance.
Introduction
With the increase in the aging population, sarcopenia has become one of the most important public health problems. It is characterized by an age-related decline in muscle mass and function, leading to a progressive loss of physical abilities, increased risk of hospitalization, and premature death (1). The main characteristics of sarcopenia include selective atrophy of fast muscle fibers, loss of motor units, and an increase in hybrid muscle fibers. Muscle strength and power decline more than muscle size, making muscle function a critical predictor of adverse outcomes (2). Despite differences in diagnostic criteria, target populations, and regional variability, the global prevalence of sarcopenia in individuals over 60 years of age is estimated to range from 10% to 27% (3, 4). Although the prevalence of sarcopenia is significantly high, it is often underdiagnosed and undertreated. Indeed, in addition to the lack of an international consensus on how to define sarcopenia, there is no accepted pharmaceutical agent to treat it, and physical activity (e.g., resistance exercise) remains the first line of treatment to improve its clinical outcomes (5). However, mounting evidence suggests that specific dietary patterns or individual nutrients may slow the progression of sarcopenia, thereby improving outcomes related to muscle mass, muscle strength, and physical function (6–8).
The most effective nutritional factors to preserve muscle mass and function include protein, vitamin D, fatty acids, and dietary antioxidants (9, 10). Given that sarcopenia is characterized by an increase in muscle protein breakdown and a decrease in muscle protein synthesis, an inadequate protein intake may aggravate the catabolic state observed in sarcopenic patients (11). Sarcopenia is also closely related to vitamin D deficiency. Recent meta-analyses have reported that co-supplementation with protein, particularly whey protein, and vitamin D increases muscle strength in patients with sarcopenia (12, 13). Current epidemiological and clinical data also show that monounsaturated fatty acids (MUFA), such as oleic acid, and omega-3 (n-3) polyunsaturated fatty acids (PUFA), such as eicosapentaenoic acid (EPA), and docosahexaenoic acid (DHA), might reduce the risk of sarcopenia due to their anti-inflammatory effects (14, 15).
Several factors contribute to sarcopenia, such as decreased levels of growth hormone (GH), insulin-like growth factor 1 (IGF-1), and testosterone, as well as immunological changes characterized by increased levels of interleukin 1 (IL-1), interleukin 6 (IL-6), tumor necrosis factor-alpha (TNF-α), and myostatin (16). Systemic chronic low-grade inflammation associated with aging (i.e., inflammaging) may contribute to the onset and development of sarcopenia. High levels of pro-inflammatory cytokines inhibit skeletal muscle protein synthesis, increase protein catabolism, and impair skeletal muscle function. Furthermore, inflammaging is closely interconnected with oxidative stress and anabolic resistance, exacerbating the loss of muscle mass, strength, and function (17, 18). Notably, sustained oxidative damage is accompanied by mitochondrial dysfunction, which enhances the loss of proteostasis in skeletal muscle cells (19, 20). Myocytes, as post-mitotic cells, are particularly susceptible to oxidative damage, leading to the accumulation of oxidatively damaged molecules. Additionally, the high oxygen consumption of skeletal muscles increases the production of mitochondria-derived reactive oxygen species (ROS). Therefore, oxidative stress contributes to sarcopenia by altering muscle fiber composition, especially affecting type II fibers, and impairing mitochondrial quality control processes, such as mitophagy, the unfolded protein response (UPR), and degradation by the ubiquitin-proteasome system. Moreover, the absence of key antioxidant enzymes, such as peroxiredoxin 6 (Prdx6), increases oxidative stress, leading to telomere shortening and exacerbating muscle atrophy (16, 21, 22).
Polyphenols are a large family of plant compounds widely present in fruits, vegetables, and certain beverages. These compounds can be broadly classified into two categories: flavonoids and nonflavonoids. However, based on their distinctive structures (e.g., number of phenol units, substituent groups, and linkages between phenol units), flavonoids are further divided into six subclasses, such as flavanones, flavonols, flavones, isoflavones, anthocyanins, and flavan-3-ols. Nonflavonoids, on the other hand, include phenolic acids, stilbenes, and lignans (23). Polyphenols exhibit a wide range of biological activities, including antioxidant, anti-inflammatory, anti-catabolic, and pro-anabolic effects, which may preserve muscle health and function (24). Although polyphenols act primarily as antioxidants and anti-inflammatory compounds, they can modulate signaling pathways involved in muscle metabolism, protein turnover, and mitochondrial biogenesis, thereby improving muscle homeostasis (25). A number of studies in in vitro and animal models provide evidence supporting the efficacy of polyphenols in attenuating age-related muscle loss and dysfunction (26). Resveratrol, a polyphenol belonging to the stilbene subclass, has been shown to improve muscle strength and mitochondrial function in rodents through activation of sirtuin 1 (SIRT1) and AMP-activated protein kinase (AMPK) pathways, demonstrating that its anti-inflammatory action may contrast sarcopenia in middle-aged mice. Furthermore, resveratrol may attenuate sarcopenia by enhancing autophagy, suggesting it could be a potential strategy for age-related sarcopenia (27–29). Several flavonoids have been demonstrated to protect against muscle atrophy and preserve muscle function in aged animals by modulating inflammatory cytokines, proteolytic enzymes, and myogenic regulatory factors (30). This systematic review and meta-analysis aims to pool current clinical evidence on the effect of polyphenol supplementation on muscle mass, muscle strength, and physical performance in individuals with sarcopenia.
Methods
Design
The search strategy, screening, and selection criteria of this systematic review and meta-analysis were conducted according to the Preferred Reporting Items for Systematic Reviews and Meta-Analyses (PRISMA) guidelines (31). The protocol for this review was registered on PROSPERO (CRD42024556145).
Search Strategy
A systematic search was performed using three databases: PubMed, Scopus, and Web of Science. The search was conducted from inception to April 2024. The following keywords and Boolean operators were used for the literature search: (“polyphenols” OR “flavonoids” OR «anthocyanins» OR «flavanols» OR «flavan-3-ols» OR «flavones» OR «flavonols» OR «flavanones» OR «flavonones» OR «isoflavones» OR “proanthocyanidins” OR “phenolic acids” OR “hydroxycinnamic acids” OR “lignans” OR “stilbenes” OR “tannins” ) AND («sarcopenia» OR «sarcopenic» OR «muscular atrophy» OR «skeletal muscle atrophy» OR «muscle mass loss» OR «skeletal muscle loss» OR «muscle mass atrophy») OR «muscle loss» OR «muscle frailty» OR «muscle wasting»). Similar queries were respectively used for controlled vocabulary search: “polyphenols” [Mesh] AND “sarcopenia” [Mesh], Indexterms “polyphenols” AND “sarcopenia”.
Eligibility Criteria
Studies were eligible for this systematic review if they met the following criteria: (1) interventional studies that evaluated the association between dietary supplementation with polyphenols or polyphenol-rich foods (for at least 4 weeks with a standardized dosage) and sarcopenia or suspected sarcopenia; (2) included only middle-aged and older subjects (≥50 years) diagnosed with sarcopenia; (3) used a sarcopenia diagnosis that included at least one component: muscle strength, muscle mass, or physical performance; and (4) were published in English. Exclusion criteria were: (1) trials without polyphenol supplementation or a described polyphenol dose; (2) studies with secondary data (conference abstracts, meta-analyses, reviews, letters, and case reports); and (3) non-human studies. The PICOS criteria (participants, interventions, comparisons, outcomes, and study design) used to define the research question are shown in Table 1.
This table shows the PICOS criteria for the inclusion of studies investigating the effects of dietary polyphenols or polyphenol-rich foods on sarcopenia and its components.
Study Selection and Data Extraction
After removing duplicate records with the reference management software EndNote X9 (Clarivate Analytics, Philadelphia, PA, USA), the titles and abstracts of retrieved articles were screened for eligibility by two researchers (AM and SD). If an abstract did not provide sufficient information for evaluation, the full text was retrieved. Disagreements were resolved by a third researcher (GS). Articles that met the eligibility criteria were selected for inclusion in the final review. A data extraction table for the included studies was then developed. The following information was extracted: first author (along with the year of publication and country of the study), study design, participant characteristics (sample size, age, sex), sarcopenia definition, type and duration of the intervention, and outcome measures.
Risk of Bias and Quality Assessment
The risk of bias of the included studies was performed using the Cochrane Risk of Bias Tool (32). The individualy quality of each domain was examined, including random sequence generation (selection bias), allocation concealment (selection bias), blinding of participants and personnel (performance bias), blinding of outcome assessment (detection bias), incomplete outcome data (attrition bias), and selective reporting (reporting bias). The quality of each study was then graded as high, moderate, or low based on the following criteria: (1) high quality if all domains were met (all sources of bias were low risk) or if one domain was of unclear risk; (2) moderate quality if one domain was not met (high risk) and one was of unclear risk, or if two domains were of unclear risk; (3) low quality if three or more domains were of unclear risk or if two or more domains were not met (high risk).
Statistical Analysis
Participants who consumed the polyphenol supplements were assigned to the active group, while those who consumed the placebo were assigned to the control group. To compare the effects of the active and placebo groups on the chosen outcomes, standardized mean differences (SMD) and the corresponding 95% confidence intervals (95% CI) were calculated. Heterogeneity among the studies was assessed using the I2 test and categorized as follows: low heterogeneity (25-49%), moderate heterogeneity (50-74%), and high heterogeneity (75-100%) (33). A random-effects model was applied in the presence of significant heterogeneity, whereas a fixed-effects model was used when heterogeneity was not significant. Sensitivity and subgroup analyses were not performed due to the small number of included studies. The meta-analysis of the included studies was carried out using Review Manager software provided by the Cochrane Collaboration. A p-value of less than 0.05 was considered to be statistically significant.
Results
Study Selection
The PRISMA flow diagram of this systematic review and meta-analysis is presented in Figure 1. A total of 414 potentially relevant articles were identified during the initial literature searches (PubMed: 251, Scopus: 236, Web of Science: 127). After removing 270 duplicates, 344 articles were screened based on titles and abstracts, and 23 full-text articles were considered for inclusion. Sixteen articles were excluded for reasons such as study protocol or design, lack of a sarcopenia diagnosis for all patients, inclusion of patients aged >50, or absence of polyphenol or polyphenol-rich food supplementation. Seven articles were included in this systematic review to examine the effects of polyphenol or polyphenol-rich food supplementation on muscle mass, strength, and physical performance in sarcopenic populations (34–40).
From 614 articles identified in PubMed, Scopus, and Web of Science, 7 were included in the qualitative analysis, and 5 in the quantitative analysis. Major exclusions were due to study protocols, no sarcopenia diagnosis, or lack of polyphenol supplementation.
Study Characteristics
The main characteristics of the included studies are shown in Table 2. The studies were published between 2007 and 2023. The countries where the studies were conducted include Canada (34), Iran (35), Japan (36, 37), Korea (38), Mexico (39), and Taipei (40). Most studies included both men and women, except for one study that included only men (35) and two studies that included only women (34, 36). Four studies were placebo-controlled trials (34, 35, 38, 39), two were randomized controlled trials (36, 37), and one was a single-arm trial (40). The sample sizes ranged from 18 to 128 subjects. The diagnostic criteria for sarcopenia included the EWGSOP (39), the AWGS (37, 40), the FNIH (38), and other criteria (34–36). Four studies used catechin, epicatechin, or epicatechin with proanthocyanidins as dietary interventions (35–37, 39), two used isoflavones (34, 40), and one used marine-derived oligomeric polyphenols (38). One study included supplementation with amino acids and polyphenols (37). The duration of the interventions ranged from 4 to 24 weeks. Three studies also incorporated exercise training plans (resistance or multi-component exercises) with or without polyphenol supplementation (35–37).
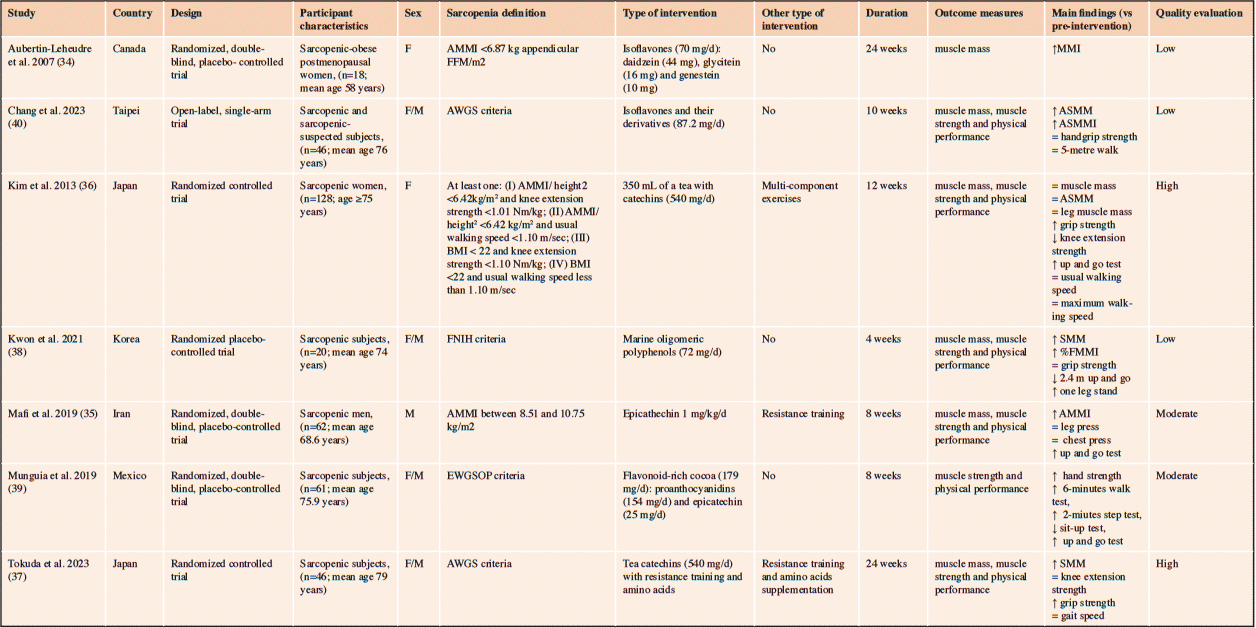
Table 2. Characteristics of the included clinical studies. This table shows the main characteristics of the included clinical studies, such as study design, participant characteristics, interventions, durations, outcome measures, and main findings
Abbreviations: FMMI, fat-free mass index; AMMI, appendicular muscle mass index; ASMM, appendicular skeletal muscle mass; ASMMI, appendicular skeletal muscle mass index; AWGS, Asian Working Group for Sarcopenia; BMI, body mass index; EWGSOP, European Working Group on Sarcopenia in Older People; FNIH, Foundation for the National Institutes of Health Sarcopenia Project; LMM, leg muscle mass; MMI, muscle mass index; SMM, skeletal muscle mass; QOL, quality of life; ↑=increase; ↓=decrease; = no difference.
The evaluation of parameters related to sarcopenia (muscle mass, muscle strength, and physical performance) and the doses of ingested polyphenols are described in detail below for each study. Aubertin-Leheudre et al. evaluated only a muscle mass parameter, the muscle mass index (MMI), calculated as appendicular fat-free mass (kg)/height (m²). The participants of this study (n=18; mean age 58 years) ingested 70 mg of isoflavones per day (active group n=12) or a placebo for 24 weeks (34). Chang et al. assessed two muscle mass parameters: appendicular skeletal muscle mass and appendicular skeletal muscle mass index (ASMMI). They also evaluated muscle strength using handgrip strength and physical performance using the 5-meter walk. The subjects (n=46; mean age 76) included in this study consumed 87.2 mg of isoflavones (active group n=46) e and their derivatives daily for 10 weeks (40). Kim et al. measured three muscle mass parameters: total muscle mass (kg), appendicular skeletal muscle mass (ASMM, kg), and leg muscle mass (kg); two muscle strength values: grip strength (kg) and knee extension strength (nm); and three physical performance results: timed up and go (s), usual walking speed (m/s), and maximum walking speed (m/s). Out of a total of 128 women included in this study, aged over 75 years, 32 participants ingested 350 mL of a tea beverage fortified with 540 mg of catechin daily for 3 months (36). Kwon et al. evaluated three muscle mass parameters: skeletal muscle mass (SMM), lean body mass (kg), and fat-free mass index (%FFMI); two muscle strength parameters: right and left grip strength; and two physical performance parameters: 2.4 m up and go, and one-leg stand. The dose of marine oligomeric polyphenols consumed by the participants (active group n=10, control group n=10; mean age 74 years) was 72 mg. Mafi et al. investigated the appendicular muscle mass index (AMMI), calculated as appendicular lean-body mass (kg)/height (m²). They also assessed two muscle strength parameters (leg press and chest press) and one physical performance parameter (timed up and go). In this study, the epicatechin group (n=17; mean age 68.6) had a daily intake of 1 mg of epicatechin per kilogram of body weight (1 mg/kg/day) for 8 weeks (35). The study conducted by Munguia et al. evaluated several muscle strength and physical performance parameters, including hand strength, 6-minute walk, 2-minute step test, sit-up test, and up-and-go test. In this randomized, double-blind, clinical trial, subjects ( n=61, mean age 75.9) were instructed to consume a flavonoid-rich cocoa beverage (n=34; epicatechin 25 mg; proanthocyanidins 154 mg) once/day for 12 weeks (39). Finally, Tokuda et al. estimated SMM (kg) to assess muscle mass, grip strength (kg), and knee extension strength (kg) to measure muscle strength, whereas gait speed (m/sec) was used to determine physical performance. Participants allocated to polyphenol supplementation (n=18; mean age 79 years) consumed 540 mg of catechins daily for 24 weeks (37).
Risk of Bias and Quality Assessment
The risks of bias in the studies are shown in Figure 2. Most domains showed a low risk of bias, including random sequence generation (71.4%), completeness of outcome data (100%), and selective reporting (85.7%). However, all studies had an unclear risk of bias for other biases (100%). Blinding of participants and personnel was low risk in five studies (71.4%), unclear in one study (14.3%), and high risk in another (14.3%). Allocation concealment was low risk in three studies (42.9%) and unclear in four studies (57.1%). Blinding of outcome assessment was low risk in two studies (28.6%), unclear in four studies (57.1%), and high risk in one study (14.3%). The overall quality of each study was also graded, showing that two studies were rated as high risk (36, 37), two as moderate (35, 39), and three as low (34, 38, 40).
Risk of bias assessment for seven studies, showing ratings across seven domains (A-G) where «+» indicates low risk, «−» indicates high risk, and «?» indicates unclear risk.
Meta-Analysis of the Effects of Polyphenols on muscle mass, strength, and physical performance
Five of the 7 studies were included in the meta-analysis as the required pooling data were available (34–36, 38, 40). These studies involved a total of 227 participants. Across the 5 studies included in the meta-analysis, measurements of muscle mass parameters were reported. The analysis found a significant difference in the effect of polyphenol supplementation on muscle mass compared to the control condition (SMD = 1.50; 95% CI: 0.26, 2.75; Z = 2.36; P = 0.02), with high heterogeneity observed among the studies (I² = 93%, P < 0.00001), as shown in Figure 3A.
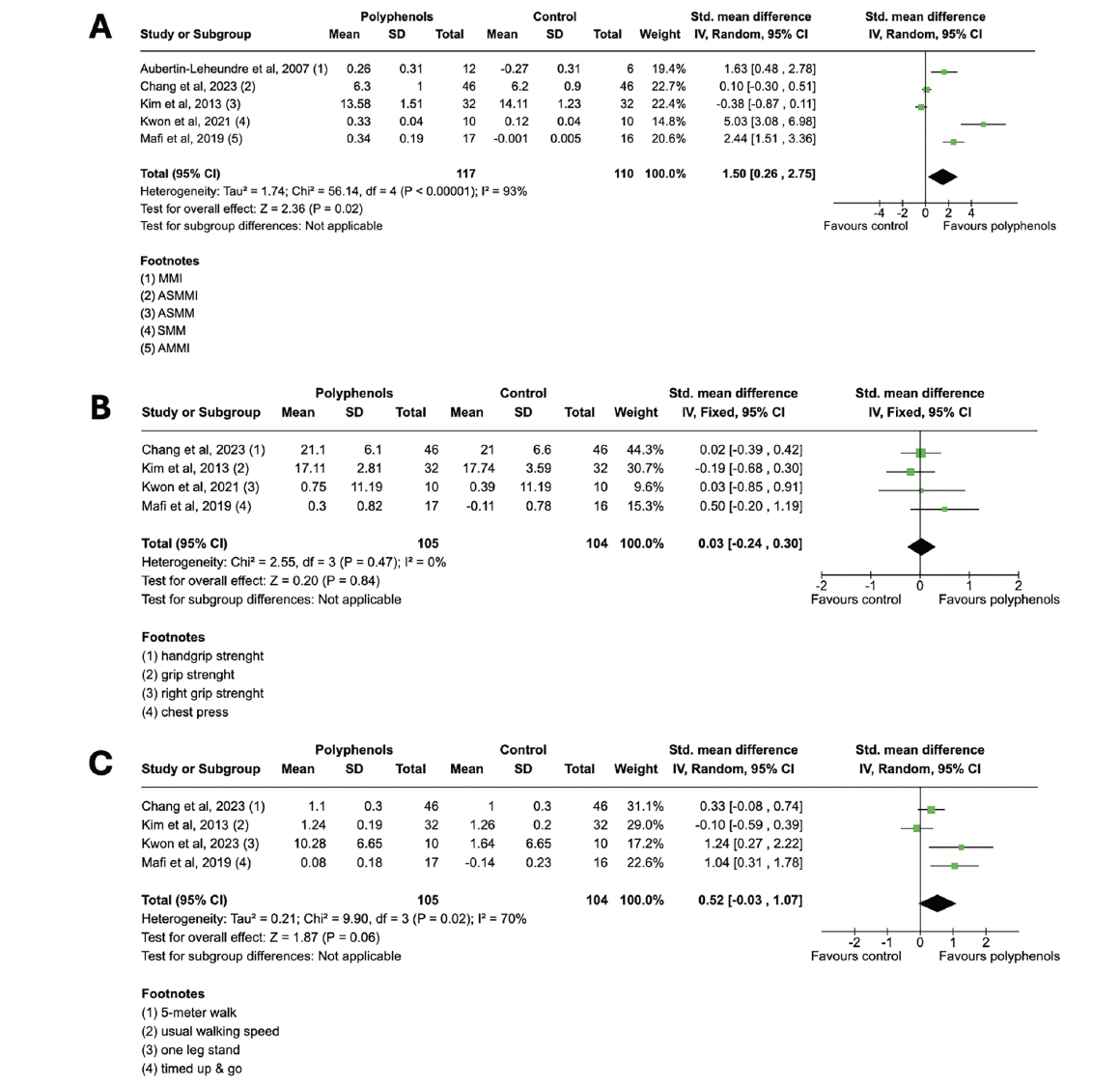
Figure 3. Pooled analysis of the impact of polyphenol supplementation on (A) muscle mass, (B) muscle strength and (C) physical performance parameters in sarcopenic subjects
Forest plots display the effects of polyphenol supplementation compared to control groups across included studies. Abbreviations: MMI, muscle mass index; ASMMI, appendicular skeletal muscle mass index; ASMM, appendicular skeletal muscle mass; SMM, skeletal muscle mass; AMMI appendicular muscle mass index
A total of 4 studies with 209 participants reported measures of muscle strength parameters. No significant difference was observed in the effect of polyphenol supplementation compared to the control condition (SMD = 0.03; 95% CI: −0.24, 0.30; Z = 0.20; P = 0.84), with no heterogeneity observed among the studies (I² = 0%, P = 0.47) (Figure 3B). Similarly, 4 studies reported gait speed as an outcome of physical performance. Despite an observable trend, combined results from the random-effects model showed no statistically significant impact on physical performance parameters following polyphenol consumption (SMD = 0.52; 95% CI: −0.03, 1.07; P = 0.06), with moderate heterogeneity among the studies (I² = 70%, P = 0.02) (Figure 3C).
Discussion
This systematic review assessed the effect of polyphenols on sarcopenia, comprising seven clinical trials with a total of 381 sarcopenic patients. From these seven studies, our meta-analysis pooled results from five clinical trials to estimate the effect of polyphenols on outcomes associated with muscle mass, while four clinical studies were meta-analyzed to evaluate the effect of polyphenols on muscle strength and physical performance. The quality of the included studies varied between low and moderate, with only two studies being assessed as high quality. Among the five studies that were meta-analyzed to estimate the effect of polyphenol supplementation on muscle mass parameters, a significant effect was found in improving indices related to muscle mass, such as MMI, ASMMI, and AMMI (34–36, 38, 40). Conversely, the four studies that were meta-analyzed to evaluate the influence of polyphenols on muscle strength parameters, such as handgrip strength and chest press, did not report significant differences between active and control groups (35, 36, 38, 40). Despite a trend toward statistical significance, the results of the meta-analysis on physical performance outcomes did not show a statistically significant influence of polyphenols on measures such as walking speed, one-leg stand, and timed up and go (35, 36, 38, 40).
As the required pooling data were not available, two studies were included in the systematic review but not in the meta-analysis (37, 39). One of these clinical studies indicates that regular consumption of a flavanol-rich cocoa beverage improves physical performance, mobility, and quality of life in older subjects. These effects were also accompanied by significant improvements in metabolic, oxidative stress, and inflammatory biomarkers (39). Indeed, flavonoids are known for their antioxidant and anti-inflammatory properties. A population-based analysis of the National Health and Nutrition Examination Survey (NHANES) found that moderate consumption of dietary flavonoids is associated with a reduced risk of frailty in middle-aged and older adults (41). Likewise, experimental studies have repeatedly demonstrated that flavonoids and their subclass compounds exert protection against muscle atrophy in various wasting conditions (42–44). Flavonoids appear to be effective in improving mitochondrial function and promoting mitochondrial biogenesis and mitophagy. Moreover, they may reverse mitochondrial dysfunction in skeletal muscle by up-regulating the peroxisome proliferator-activated receptor gamma coactivator-1 alpha (PGC-1α) signaling pathway (45, 46). The second study that was not included in the meta-analysis used tea catechins combined with essential amino acids and resistance exercise to evaluate the effects of these compounds on skeletal muscle mass in older adults with sarcopenia. The results of this study suggest that ingestion of tea catechins improved skeletal muscle mass more than exercise alone in older subject with sarcopenia (37). Catechins belong to the flavanol subclass of flavonoids, and tea, especially green tea, contains high levels of polyphenolic catechins. The findings of the study conducted by Tokuda et al., included in this systematic review, are consistent with previous experimental evidence showing that green tea polyphenols preserve skeletal muscle atrophy in a rat model of sarcopenia by attenuating protein degradation and increasing the expression of anabolic factors (47). Another two studies included in this review evaluated the effects of catechins combined with physical exercise, demonstrating that these two interventions together improve walking ability and muscle mass in sarcopenic subjects (35, 36).
Isoflavones, flavonoid compounds commonly present in soy foods and legumes, were used in two clinical studies included in this review (34, 40). Several experimental studies indicate that isoflavones, such as genistein and daidzein, may alleviate skeletal muscle atrophy, prevent protein degradation, promote myogenic differentiation, and induce myotube hypertrophy (48–51). A further clinical study included in this review shows that marine polyphenols may improve skeletal muscle mass, fat-free mass, and physical ability (one-leg stand) in sarcopenic individuals (38). Given that inflammation contributes to the development of sarcopenia, these findings are consistent with in vitro evidence showing that marine polyphenols present in brown seaweeds inhibit the production of inflammatory cytokines in skeletal muscle cells (52).
It is important to highlight that the effects of polyphenols are not only dependent on their intake but also on their bioavailability, which is influenced by absorption, metabolism, and tissue disposition. Moreover, the rate of absorption of these compounds is influenced by the intestinal microbiota, which, in older subjects, is characterized by a marked decrease in microbial diversity and dysbiosis (53). The studies included in this review did not measure the bioavailability and absorption rates of polyphenols in their cohorts, and therefore, the results should be interpreted with caution. Most of the included studies used a wide variety of dosages of polyphenols and different treatment durations. Moreover, a preliminary dietary assessment was performed and reported in only one study (40). This assessment is crucial to estimate the polyphenol content in the habitual diet.
Many different diagnostic criteria for sarcopenia were used in the included studies, such as EWGSOP (European Working Group on Sarcopenia in Older People), AWGS (Asian Working Group for Sarcopenia), and FNIH (Foundation for the National Institutes of Health Sarcopenia Project). Two studies used only AMMI and FMMI to diagnose sarcopenia in their cohorts (34, 35). The differences in the criteria used to define sarcopenia in the included articles may have influenced the level of heterogeneity observed in this meta-analysis.
Strengths and Limitations
This review has several strengths. First, to our knowledge, this is the first article to systematically review available clinical evidence on the effects of polyphenols in sarcopenic subjects. Second, a robust literature search protocol was developed, using specific eligibility criteria and controlled vocabulary queries to comprehensively retrieve relevant studies. Third, risk of bias and quality assessment were performed to evaluate the methodological quality of the included studies. However, this review has also some limitations. The number of studies included in the systematic review and meta-analysis was limited. There was a substantial level of heterogeneity in the pooled analysis of the impact of polyphenols on muscle mass and physical performance. Subgroup analyses were not conducted to reduce heterogeneity due to the small number of included studies.
Conclusions
Although the available data are still insufficient to confirm that polyphenols may have clinical efficacy against sarcopenia, the findings of this systematic review and meta-analysis seem to provide evidence that these compounds have the potential to counteract some of the main effects of sarcopenia, particularly muscle mass. However, it is essential to interpret these data with caution. Future research needs more rigorously designed clinical studies, including well-powered and long-term trials to evaluate optimal dosage and treatment duration. Additionally, given the polyphenol content in habitual diets, preliminary dietary intake assessments and measurements of intake biomarkers are needed to determine the specific effects of polyphenols on sarcopenia.
Author contribution: AM and SD: conception and design; AM and SD acquisition of data, analysis and interpretation of data; AM, GS, and SD: drafting the article and revising it critically for important intellectual content.
Funding: No source of funding is associated with this article. Open access funding provided by Università degli Studi del Molise within the CRUI-CARE Agreement.
Data Availability: Upon request.
Competing interests: The authors declare no conflict of interest.
Ethical Approval: Not applicable.
Open Access: This article is distributed under the terms of the Creative Commons Attribution 4.0 International License (http://creativecommons.org/licenses/by/4.0/), which permits use, duplication, adaptation, distribution and reproduction in any medium or format, as long as you give appropriate credit to the original author(s) and the source, provide a link to the Creative Commons license and indicate if changes were made.
References
1. Larsson L, Degens H, Li M, et al. Sarcopenia: Aging-Related Loss of Muscle Mass and Function. Physiol Rev 2019;99:427; doi: 10.1152/physrev.00061.2017.
2. Cruz-Jentoft AJ, Bahat G, Bauer J, et al. Sarcopenia: revised European consensus on definition and diagnosis. Age Ageing 2019;48:601; doi: 10.1093/ageing/afz046
3. Petermann-Rocha F, Balntzi V, Gray SR, et al. Global prevalence of sarcopenia and severe sarcopenia: a systematic review and meta-analysis. J Cachexia Sarcopenia Muscle 2022;13:86–99; doi: 10.1002/jcsm.12783
4. Shafiee G, Keshtkar A, Soltani A, Ahadi Z, Larijani B, Heshmat R. Prevalence of sarcopenia in the world: a systematic review and meta- analysis of general population studies. J Diabetes Metab Disord 2017;16; doi: 10.1186/s40200-017-0302-x.
5. Coletta G, Phillips SM. An elusive consensus definition of sarcopenia impedes research and clinical treatment: A narrative review. Ageing Res. Rev.2023, 86: 101883; doi: 10.1016/j.arr.2023.101883
6. Davinelli S, Corbi G, Scapagnini G. Frailty syndrome: A target for functional nutrients? Mech Ageing Dev 2021;195; doi: 10.1016/j.mad.2021.111441
7. Bloom I, Shand C, Cooper C, Robinson S, Baird J. Diet Quality and Sarcopenia in Older Adults: A Systematic Review. Nutrients 2018;10; doi: 10.1016/j.mad.2021.111441
8. Robinson S, Granic A, Cruz-Jentoft AJ, Sayer AA. The role of nutrition in the prevention of sarcopenia. Am J Clin Nutr 2023;118:852–864; doi: 10.1016/j.ajcnut.2023.08.015
9. Robinson SM, Reginster JY, Rizzoli R, et al. Does nutrition play a role in the prevention and management of sarcopenia? Clin Nutr 2018;37:1121–1132; doi: 10.1016/j.clnu.2017.08.016.
10. Cesare MM, Felice F, Santini V, Di Stefano R. Antioxidants in Sport Sarcopenia. Nutrients 2020;12:1–20; doi: 10.3390/nu12092869
11. Coelho-Junior HJ, Calvani R, Azzolino D, et al. Protein Intake and Sarcopenia in Older Adults: A Systematic Review and Meta-Analysis. Int J Environ Res Public Health 2022;19; doi: 10.3390/ijerph19148718
12. Gkekas NK, Anagnostis P, Paraschou V, et al. The effect of vitamin D plus protein supplementation on sarcopenia: A systematic review and meta-analysis of randomized controlled trials. Maturitas 2021;145:56–63; doi:10.1016/j.maturitas.2021.01.002
13. Nasimi N, Sohrabi Z, Nunes EA, et al. Whey Protein Supplementation with or without Vitamin D on Sarcopenia-Related Measures: A Systematic Review and Meta-Analysis. Adv Nutr 2023;14:762–773; doi: 10.1016/j.advnut.2023.05.011
14. Ali S, Corbi G, Medoro A, Intrieri M, Scapagnini G, Davinelli S. Relationship between monounsaturated fatty acids and sarcopenia: a systematic review and meta-analysis of observational studies. Aging Clin Exp Res 2023;35:1823–1834; doi: 10.1007/s40520-023-02465-0
15. Bird JK, Troesch B, Warnke I, Calder PC. The effect of long chain omega-3 polyunsaturated fatty acids on muscle mass and function in sarcopenia: A scoping systematic review and meta-analysis. Clin Nutr ESPEN 2021;46:73–86; doi: 10.1016/j.clnesp.2021.10.011
16. Wiedmer P, Jung T, Castro JP, et al. Sarcopenia – Molecular mechanisms and open questions. Ageing Res Rev 2021;65; doi: 10.1016/j.arr.2020.101200
17. Pan L, Xie W, Fu X, et al. Inflammation and sarcopenia: A focus on circulating inflammatory cytokines. Exp Gerontol 2021;154:111544; doi: 10.1016/j.exger.2021.111544
18. Meng SJ, Yu LJ. Oxidative Stress, Molecular Inflammation and Sarcopenia. Int J Mol Sci 2010;11:1509–1526; doi: 10.3390/ijms11041509
19. Fernando R, Drescher C, Nowotny K, Grune T, Castro JP. Impaired proteostasis during skeletal muscle aging. Free Radic Biol Med 2019;132:58–66; doi:10.1016/j.freeradbiomed.2018.08.037
20. Ferri E, Marzetti E, Calvani R, Picca A, Cesari M, Arosio B. Role of Age-Related Mitochondrial Dysfunction in Sarcopenia. Int J Mol Sci;2020;21:5236; doi: 10.3390/ijms21155236
21. Pacifici F, Della-Morte D, Piermarini F, et al. Prdx6 Plays a Main Role in the Crosstalk Between Aging and Metabolic Sarcopenia. Antioxidants (Basel) 2020;9:329; doi: 10.3390/antiox9040329.
22. Scicchitano BM, Pelosi L, Sica G, Musarò A. The physiopathologic role of oxidative stress in skeletal muscle. Mech Ageing Dev 2018;170:37–44; doi: 10.1016/j.mad.2017.08.009
23. Durazzo A, Lucarini M, Souto EB, et al. Polyphenols: A concise overview on the chemistry, occurrence, and human health. Phytother Res 2019;33:2221–2243; doi: 10.1002/ptr.6419
24. Salucci S, Falcieri E. Polyphenols and their potential role in preventing skeletal muscle atrophy. Nutr Res 2020;74:10–22; doi: 10.1016/j.nutres.2019.11.004
25. Nikawa T, Ulla A, Sakakibara I. Polyphenols and Their Effects on Muscle Atrophy and Muscle Health. Molecules 2021;26; doi: 10.3390/molecules26164887
26. Felice F, Cesare MM, Fredianelli L, et al. Effect of Tomato Peel Extract Grown under Drought Stress Condition in a Sarcopenia Model. Molecules 2022;27; doi: 10.3390/molecules27082563
27. Liao ZY, Chen JL, Xiao MH, et al. The effect of exercise, resveratrol or their combination on Sarcopenia in aged rats via regulation of AMPK/Sirt1 pathway. Exp Gerontol 2017;98:177–183; doi: 10.1016/j.exger.2017.08.032
28. Hosoda R, Nakashima R, Yano M, et al. Resveratrol, a SIRT1 activator, attenuates aging-associated alterations in skeletal muscle and heart in mice. J Pharmacol Sci 2023;152:112–122; doi: 10.1016/j.jphs.2023.04.001
29. Sirago G, Toniolo L, Crea E, Giacomello E. A short-term treatment with resveratrol improves the inflammatory conditions of Middle-aged mice skeletal muscles. Int J Food Sci Nutr 2022;73:630–637; doi:10.1080/09637486.2022.2027889
30. Kim C, Hwang JK. Flavonoids: nutraceutical potential for counteracting muscle atrophy. Food Sci Biotechnol 2020;29:1619; doi: 10.1007/s10068-020-00816-5
31. Page MJ, McKenzie JE, Bossuyt PM, et al. The PRISMA 2020 statement: an updated guideline for reporting systematic reviews. Syst Rev 2021;10:1–11; doi: 10.1186/s13643-021-01626-4
32. Higgins JPT, Altman DG, Gøtzsche PC, et al. The Cochrane Collaboration’s tool for assessing risk of bias in randomised trials. BMJ 2011;343; doi: 10.1136/bmj.d5928
33. Thorlund K, Imberger G, Johnston BC, et al. Evolution of heterogeneity (I2) estimates and their 95% confidence intervals in large meta-analyses. PLoS One 2012;7:e39471; doi: 10.1371/journal.pone.0039471
34. Aubertin-Leheudre M, Lord C, Khalil A, Dionne IJ. Six months of isoflavone supplement increases fat-free mass in obese-sarcopenic postmenopausal women: a randomized double-blind controlled trial. Eur J Clin Nutr 2007;61:1442–1444; doi: 10.1038/sj.ejcn.1602695
35. Mafi F, Biglari S, Afousi AG, Gaeini AA. Improvement in Skeletal Muscle Strength and Plasma Levels of Follistatin and Myostatin Induced by an 8-Week Resistance Training and Epicatechin Supplementation in Sarcopenic Older Adults. J Aging Phys Act 2019;27:384–391; doi: 10.1123/japa.2017-0389
36. Kim H, Suzuki T, Saito K, et al. Effects of exercise and tea catechins on muscle mass, strength and walking ability in community-dwelling elderly Japanese sarcopenic women: A randomized controlled trial. Geriatr Gerontol Int 2013;13:458–465; doi:10.1111/j.1447-0594.2012.00923.x
37. Tokuda Y, Mori H. Essential Amino Acid and Tea Catechin Supplementation after Resistance Exercise Improves Skeletal Muscle Mass in Older Adults with Sarcopenia: An Open-Label, Pilot, Randomized Controlled Trial. J Am Nutr Assoc 2023;42:255–262; doi:10.1080/07315724.2022.2025546
38. Kwon IS, Park DS, Shin HC, Seok MG, Oh JK. Effects of marine oligomeric polyphenols on body composition and physical ability of elderly individuals with sarcopenia: a pilot study. Phys Act Nutr 2021;25:1–7; doi: 10.20463/pan.2021.0014
39. Munguia L, Rubio-Gayosso I, Ramirez-Sanchez I, et al. High flavonoid cocoa supplement ameliorates plasma oxidative stress and inflammation levelswhile improving mobility and quality of life in older subjects: A double-blind randomized clinical trial. Journals Gerontol – Ser A Biol Sci Med Sci 2019;74:1620–1627; doi: 10.1093/gerona/glz107
40. Chang SS, Chen LH, Huang KC, et al. Plant-based polyphenol rich protein supplementation attenuated skeletal muscle loss and lowered the LDL level via gut microbiota remodeling in Taiwan’s community-dwelling elderly. Food Funct 2023;14:9407–9418; doi: 10.1039/d3fo02766j
41. Guo HJ, Ye YL, Cao R, Yu TH, He Q. Association between Dietary Flavonoid Intake and the Likelihood of Frailty in Middle-Aged and Older Adults: A Population-Based Analysis from the National Health and Nutrition Examination Survey (NHANES). J Frailty Aging 2024;1–10; doi: 10.14283/jfa.2024.40
42. Mukai R, Matsui N, Fujikura Y, et al. Preventive effect of dietary quercetin on disuse muscle atrophy by targeting mitochondria in denervated mice. J Nutr Biochem 2016;31:67–76; doi: 10.1016/j.jnutbio.2016.02.001
43. Kim Y, Kim CS, Joe Y, Chung HT, Ha TY, Yu R. Quercetin Reduces Tumor Necrosis Factor Alpha-Induced Muscle Atrophy by Upregulation of Heme Oxygenase-1. J Med Food 2018;21:551–559; doi: 10.1089/jmf.2017.4108
44. Chan ST, Chuang CH, Lin YC, Liao JW, Lii CK, Yeh SL. Quercetin enhances the antitumor effect of trichostatin A and suppresses muscle wasting in tumor-bearing mice. Food Funct 2018;9:871–879; doi: 10.1039/c7fo01444a
45. Davinelli S, De Stefani D, De Vivo I, Scapagnini G. Polyphenols as Caloric Restriction Mimetics Regulating Mitochondrial Biogenesis and Mitophagy. Trends Endocrinol. Metab 2020;31: 536–550; doi: 10.1016/j.tem.2020.02.011
46. Huang Y, Chen K, Ren Q, et al. Dihydromyricetin Attenuates Dexamethasone-Induced Muscle Atrophy by Improving Mitochondrial Function via the PGC-1α Pathway. Cell Physiol Biochem 2018;49:758–779; doi: 10.1159/000493040
47. Meador BM, Mirz KA, Tian M, et al. The Green Tea Polyphenol Epigallocatechin-3-Gallate (EGCg) Attenuates Skeletal Muscle Atrophy in a Rat Model of Sarcopenia. J Frailty Aging 2015;4:1–7; doi: 10.14283/jfa.2015.58
48. Zhang H, Chi M, Chen L, et al. Daidzein alleviates cisplatin-induced muscle atrophy by regulating Glut4/AMPK/FoxO pathway. Phytother Res 2021;35:4363–4376; doi: 10.1002/ptr.7132
49. Ogawa M, Kitano T, Kawata N, et al. Daidzein down-regulates ubiquitin-specific protease 19 expression through estrogen receptor β and increases skeletal muscle mass in young female mice. J Nutr Biochem 2017;49:63–70; doi: 10.1016/j.jnutbio.2017.07.017
50. Lee SJ, Vuong TA, Go GY, et al. An isoflavone compound daidzein elicits myoblast differentiation and myotube growth. J Funct Foods 2017;38:438–446; https://doi.org/10.1016/j.jff.2017.09.016
51. Khairallah RJ, O’Shea KM, Ward CW, Butteiger DN, Mukherjea R, Krul ES. Chronic dietary supplementation with soy protein improves muscle function in rats. PLoS One 2017;12; doi: 10.1371/journal.pone.0189246
52. Kang S ym, Kim E, Kang I, Lee M, Lee Y. Anti-Diabetic Effects and Anti-Inflammatory Effects of Laminaria japonica and Hizikia fusiforme in Skeletal Muscle: In Vitro and In Vivo Model. Nutrients 2018;10; doi: 10.3390/nu10040491
53. Davinelli S, Scapagnini G. Interactions between dietary polyphenols and aging gut microbiota: A review. BioFactors 2022;48:274–284; doi: 10.1002/biof.1785
The Author(s) 2024